Arizona State University and Sandia National Laboratories advance quantum computing with photons
In the global race to bring quantum computing to the masses, a joint project between Arizona State University’s Quantum Collaborative and Sandia National Laboratories (Sandia) is charting new ground, unlocking the potential of photons, and pioneering the development of photonic quantum computing.
Funded through Sandia’s Laboratory Directed Research and Development (LDRD), the project is led by Nils Otterstrom, a Sandia physicist specializing in integrated photonics, and Dr. Joseph Lukens, Associate Professor of Electrical and Computer Engineering at Purdue University and former Senior Director of Quantum Networking at ASU.
Through this collaboration, the researchers are charting new territory through experiments and demonstrations to develop the first generation of ubiquitous quantum computers and networks.
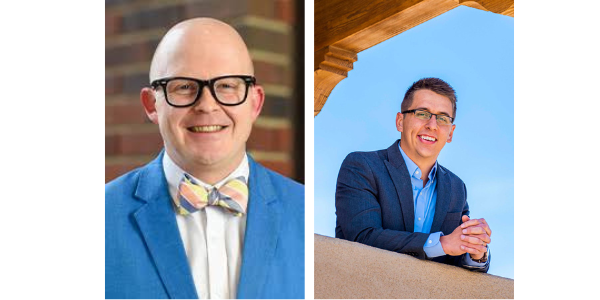
Pictured: Joseph Lukens and Nils Otterstrom
Solving historical challenges: putting photonic qubits onto microchips
The project combines Lukens’ years of research expertise in fiber-optic frequency-bin encoding, a subfield of quantum networking and communications encoding information in photons, with Sandia’s advanced capabilities in photonic integrated circuits (PICs).
“There are many amazing things that one can do with photons, especially for quantum computing,” says Lukens.
While Lukens has explored the possibilities of leveraging photonic qubits for quantum networking and computing through his experiments on optical tables, the size (up to a few meters in width), expense, and labor required to run so-called “tabletop” systems do not make them a feasible hardware option for scaling quantum computing networks.
Additionally, the inherent properties of photons as particles of light (e.g., moving at the speed of light) versus electrons, which can be contained in electrical circuits and are used in classical computers, for example, present challenges and have slowed research into their applications in quantum computing.
As such, quantum computing research has mainly focused on leveraging other platforms over photons, such as trapped-ions, cold atoms, and superconducting circuits, to build these systems – until now.
Quantum Collaborative and Sandia – “a perfect match”
Condensing a system the size of an optical table onto a chip, which is only a few square centimeters, is no easy feat. But for the task, Lukens found the right partner in Sandia.
“For photons, an exciting way to make these devices practical is through PICs,” says Lukens. “Just as we have computer chips based on electrons for the myriad of electronic devices today, we can build similar chips for photons.”
Turning Lukens’ vision into reality with initial seed funding provided by Quantum Collaborative at ASU, Lukens and Sandia began to build unique frequency coding capabilities onto chips; and did so successfully.
“Sandia really helped me learn how to test these chips and implement them in my own lab,” says Lukens. After experiencing initial success translating his proof-of-principle experiments from optical tables onto chips, the team secured additional funding by winning one of Sandia’s Grand Challenge LDRD projects to take the tests further.
Streamlining fabrication and reducing error in chip design
Collaborating with Sandia, a leading foundry for building both electronic and integrated circuits, allowed Lukens to surmount one of the biggest problems of building PICs – the long timeline and tedious development process presented by regimented foundry workflows.
Fabricating chips is not an overnight process. After finalizing a design, it takes several months before the chip is returned to the researchers. The stakes are high to get designs accurate before production since chip errors are not discoverable until the completed chips are built and tested.
“Thankfully, working with Sandia, we can mitigate these issues,” says Lukens. “I’m working with a brilliant group of scientists who are careful and help minimize errors in the critical design phase.”
After perfecting a PIC’s design, its controllability makes designs easily mass-produced with consistently reliable production, making it an ideal platform for scaling quantum computing networks.
How photons can help reach quantum supremacy
Researchers are working towards achieving quantum ubiquity. While quantum computers are beginning to solve problems not feasible for classical computers, scaling quantum computing networks remains an industry challenge that Lukens hopes PICs can help solve.
“Quantum networks are an enabler of everything else that quantum computing can provide,” says Lukens. “If you have a single quantum computer, maybe you’re limited on how much computing power it has. But with a network, you can connect multiple quantum computers to harness the power of entanglement.”
As another example, Lukens points to quantum sensors for detecting magnetic fields or gravitational waves.
“Suppose you have a quantum sensor that can detect some quantity of interest, like a small magnetic field or gravitational wave – if you connect multiple devices through entanglement on the quantum network, you can achieve better sensitivity. So quantum networks are enablers.”
PICs can also help address the high-energy demands of scaling quantum platforms since photonic qubits do not require a near-zero-degree temperature like most matter-based qubits to maintain their quantum state.
Exciting prospects for photonic quantum computing applications
With the new funding, Lukens and his team will continue researching interesting scientific applications of photonic quantum computing; from solving complicated atomic or quantum-level dynamic systems to quantum chemistry, the opportunities are boundless.
“What better way to solve an unknown quantum problem than with a well-controlled quantum system?” Lukens says.
Photonic quantum computing may also be the key to solving another industry challenge – adopting quantum security and safeguarding current cybersecurity encryption methods against powerful quantum algorithms.
“Quantum computers will eventually be able to break standard encryption, and one of our mission-driven applications for developing PICs is to make sure we don’t get caught flat-footed once that happens.”
Next steps for the PIC project
The PIC project spans both students and faculty across ASU and Sandia, bridging academic and professional environments and giving students critical exposure to the fabrication process from start to finish.
“It’s exciting for students to be exposed to both environments because they are the future and ultimately the ones that will be involved in developing and building useful quantum computers,” says Lukens.
“The next generation will be critical to making quantum computing a reality and using it once it’s available.”
Lukens recently transferred to Purdue University and looks forward to sharing the lessons he learned at ASU with his new students and colleagues.
Inspiring the next generation of quantum innovators
Fundamental knowledge and mastery of mathematics are crucial skills for success in quantum computing. But when it comes to getting involved, Lukens is encouraging to everyone.
“The goal of quantum information science is to be useful to people, but it is often presented as a mysterious, confusing thing,” says Lukens. “You don’t have to fully understand quantum mechanics to use it or even be good at it.”